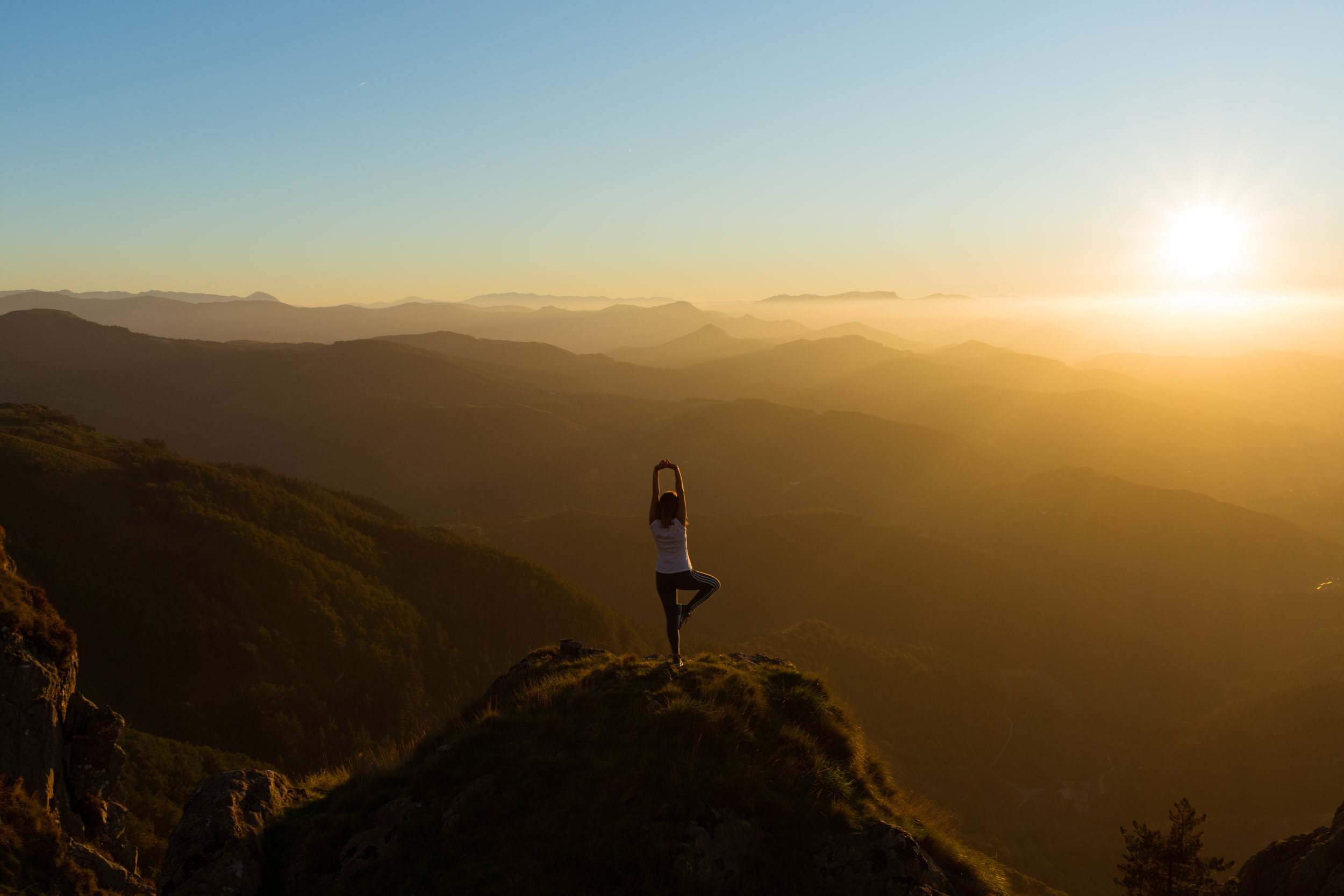
RECENT
Human Optimization Technologies: Exploring the Cutting Edge of Biohacking Tools
In today's rapidly advancing world, human optimization technologies are emerging as powerful tools for enhancing physical and mental performance, improving overall well-being, and pushing the boundaries of human potential. This article delves into the cutting-edge field of human optimization technologies, exploring the latest innovations, their potential benefits, and the exciting possibilities they offer.
In today's rapidly advancing world, human optimization technologies are emerging as powerful tools for enhancing physical and mental performance, improving overall well-being, and pushing the boundaries of human potential. This article delves into the cutting-edge field of human optimization technologies, exploring the latest innovations, their potential benefits, and the exciting possibilities they offer.
Understanding Human Optimization Technologies
Human optimization technologies encompass a wide range of tools and techniques designed to optimize various aspects of human performance and well-being. These technologies leverage scientific advancements and innovative approaches to enhance physical abilities, cognitive function, longevity, and overall quality of life. Here are some key areas of human optimization technologies:
Nutrigenomics
Nutrigenomics explores the relationship between nutrition and gene expression. By analyzing an individual's genetic makeup, nutrigenomics helps personalize dietary recommendations to optimize health and performance.
Wearable Technology
Wearable devices, such as fitness trackers and smartwatches, have gained popularity for monitoring and optimizing physical activity, sleep patterns, heart rate, and other biometric data. These devices provide real-time feedback and insights to support performance enhancement and overall well-being.
Brain-Computer Interfaces (BCIs)
BCIs establish direct communication pathways between the brain and external devices, enabling individuals to control devices using their thoughts. BCIs hold potential in assisting individuals with disabilities and unlocking new possibilities for human-machine interaction.
Virtual Reality (VR) and Augmented Reality (AR)
VR and AR technologies create immersive virtual environments or overlay digital information onto the real world. These technologies offer applications in various domains, including training, therapy, entertainment, and cognitive enhancement.
Genetic Engineering and Gene Editing
Advancements in genetic engineering and gene editing techniques, such as CRISPR-Cas9, allow for targeted modifications to the human genome. These technologies hold promise in treating genetic disorders, enhancing traits, and extending human lifespan.
Prominent Human Optimization Technologies
In recent years, several human optimization technologies have gained significant attention for their potential to enhance human performance and well-being. Here are some notable examples:
Transcranial Magnetic Stimulation (TMS)
TMS involves the use of electromagnetic fields to stimulate specific regions of the brain. It has shown promise in treating depression, enhancing cognitive function, and modulating brain activity.
Cryonics
Cryonics is the practice of preserving a person's body at ultra-low temperatures after death in the hope of future revival using advanced technologies. Advocates believe that cryonics may offer a pathway to extended life or potential rejuvenation in the future.
Biofeedback Training
Biofeedback training utilizes sensors to measure physiological parameters, such as heart rate variability, brainwave activity, or skin conductance. By providing real-time feedback, individuals can learn to control these bodily functions, leading to improved self-regulation and performance.
Bioprinting
Bioprinting technology enables the fabrication of three-dimensional biological structures, such as organs and tissues, using a combination of cells, biomaterials, and bioinks. This technology holds promise in the field of regenerative medicine and organ transplantation.
Nanotechnology
Nanotechnology involves manipulating matter at the nanoscale to develop new materials and devices with unique properties. In the field of human optimization, nanotechnology has the potential to revolutionize drug delivery systems, tissue engineering, and diagnostics.
Optogenetics
Optogenetics is a technique that combines genetic engineering and light stimulation to control and manipulate the activity of specific cells in the brain. It offers precise control over neural circuits, allowing researchers to understand brain function and potentially develop therapeutic interventions for neurological conditions.
Neurostimulation
Neurostimulation involves applying electrical or magnetic stimuli to specific areas of the brain or peripheral nerves. This technique has shown promise in treating conditions like Parkinson's disease, epilepsy, and chronic pain. It also holds potential for cognitive enhancement and mood regulation.
Photobiomodulation
Photobiomodulation (PBM) utilizes low-level light therapy to stimulate cellular function and promote tissue repair. It has shown promise in various applications, including wound healing, pain management, and cognitive enhancement.
Benefits and Considerations
Human optimization technologies offer a range of potential benefits for individuals seeking to enhance their performance and well-being. These technologies can help improve physical abilities, cognitive function, longevity, and overall quality of life. However, it's important to consider certain factors:
Individual Variability
Different technologies may have varying effects on individuals due to genetic, physiological, and lifestyle differences. What works for one person may not work the same way for another. Personalization and customization are key considerations in maximizing the benefits of these technologies.
Ethical and Safety Considerations
As human optimization technologies push the boundaries of what is possible, ethical questions arise regarding privacy, consent, and potential unintended consequences. Safety and ethical guidelines must be established to ensure responsible and beneficial use of these technologies.
Long-Term Implications
The long-term effects and potential risks associated with some human optimization technologies are still being studied. It's important to consider the balance between short-term benefits and long-term implications when adopting these technologies.
In the second part of this article, we will explore additional human optimization technologies, discuss their applications, and delve into the future prospects of this exciting field.
Future Prospects and Considerations
The field of human optimization technologies is continuously evolving, with exciting prospects on the horizon. Here are some future directions and considerations:
Personalized Precision Medicine
Advances in genomic sequencing and personalized medicine will enable more targeted and tailored approaches to human optimization. Understanding an individual's unique genetic makeup can guide interventions for optimal health, performance, and disease prevention.
Brain-Computer Interface Advancements
Continued advancements in brain-computer interfaces (BCIs) hold the potential for groundbreaking applications, such as restoring motor function in paralysis, enhancing memory and cognition, and enabling direct brain-to-brain communication.
Artificial Intelligence Integration
The integration of artificial intelligence (AI) algorithms and machine learning with human optimization technologies can enhance data analysis, prediction modeling, and personalized recommendations. AI-driven systems can optimize interventions based on individual responses and continuously adapt to achieve the best outcomes.
Ethical and Social Implications
As human optimization technologies continue to advance, it is crucial to address ethical and social implications. Discussions around equity, accessibility, privacy, and potential disparities arising from the use of these technologies are essential to ensure responsible and equitable implementation.
Regulation and Safety
As with any emerging field, regulation and safety guidelines need to be established to ensure the responsible development, deployment, and monitoring of human optimization technologies. Collaboration between researchers, industry, and regulatory bodies is vital to navigate the evolving landscape of these technologies.
Conclusion
Human optimization technologies represent the forefront of scientific advancements aimed at enhancing human performance, well-being, and longevity. From brain-computer interfaces and genetic engineering to 3D bioprinting and neurostimulation, these cutting-edge tools offer tremendous potential for improving various aspects of human existence.
As we explore the applications and future prospects of human optimization technologies, it is crucial to balance innovation with ethical considerations, individual variability, and long-term implications. Responsible development, personalized approaches, and a strong regulatory framework will be key to harnessing the full potential of these technologies for the benefit of individuals and society as a whole.
Neurofeedback for Anxiety: Training the Brain to Reduce Anxiety Symptoms
While traditional treatments such as therapy and medication can be effective, emerging techniques like neurofeedback offer a promising alternative. Neurofeedback harnesses the brain's capacity for self-regulation and has shown potential in reducing anxiety symptoms. This article explores the concept of neurofeedback for anxiety, its underlying principles, and its potential applications in anxiety management.
Anxiety disorders affect millions of people worldwide, impacting their daily lives and overall well-being. While traditional treatments such as therapy and medication can be effective, emerging techniques like neurofeedback offer a promising alternative. Neurofeedback harnesses the brain's capacity for self-regulation and has shown potential in reducing anxiety symptoms. This article explores the concept of neurofeedback for anxiety, its underlying principles, and its potential applications in anxiety management.
Understanding Anxiety and its Impact
To understand the potential of neurofeedback in anxiety management, it is important to grasp the nature of anxiety and its impact on individuals. Here are key points to consider:
Definition of Anxiety
Anxiety refers to a persistent feeling of fear, worry, or unease that can significantly impact daily functioning and quality of life. It is characterized by excessive and irrational thoughts, physical symptoms such as rapid heartbeat and sweating, and avoidance behaviors.
Types of Anxiety Disorders
Anxiety disorders encompass a range of conditions, including generalized anxiety disorder (GAD), panic disorder, social anxiety disorder, and specific phobias. Each type of anxiety disorder has its own distinct symptoms and triggers.
Impact on Daily Life
Anxiety can have a profound impact on various aspects of an individual's life, including relationships, work or school performance, and overall emotional well-being. It can lead to avoidance behaviors, impaired concentration, and reduced quality of life.
Neurofeedback: Principles and Mechanisms
Neurofeedback is a non-invasive technique that aims to train the brain to self-regulate and reduce anxiety symptoms. Here are some key principles and mechanisms of neurofeedback:
Self-Regulation
Neurofeedback operates on the principle that the brain can learn to regulate its own activity. By providing real-time feedback on brainwave patterns, individuals can gain awareness and control over their brain's functioning, leading to improved self-regulation.
Brainwave Activity
Neurofeedback primarily focuses on specific brainwave frequencies associated with anxiety. Alpha and theta frequencies are often targeted to promote relaxation and reduce hyperarousal commonly seen in anxiety disorders.
Operant Conditioning
Neurofeedback utilizes the principles of operant conditioning to reinforce desired brainwave patterns. Through repeated training sessions, individuals learn to produce and maintain specific brainwave states associated with reduced anxiety.
Feedback Mechanism
During a neurofeedback session, individuals receive real-time feedback on their brainwave activity through visual or auditory cues. This feedback allows them to observe the changes in their brainwave patterns and adjust their mental state accordingly.
Neuroplasticity
Neurofeedback capitalizes on the brain's neuroplasticity, its ability to reorganize and form new connections. By repeatedly engaging in neurofeedback training, individuals can potentially rewire their neural networks and establish more adaptive patterns of brain activity.
Neurofeedback for Anxiety: Evidence and Benefits
Numerous studies have explored the efficacy of neurofeedback in anxiety management. While the field is still evolving, initial findings are promising. Here are key findings and benefits of neurofeedback for anxiety:
Symptom Reduction
Research suggests that neurofeedback can lead to significant reductions in anxiety symptoms, including excessive worry, panic attacks, and social anxiety. Individuals report improved emotional well-being and a greater sense of control over their anxiety.
Generalization of Skills
The skills learned through neurofeedback training have the potential to generalize beyond the training sessions. Individuals may experience reduced anxiety symptoms in real-life situations, demonstrating the transferability of self-regulation skills.
Non-Invasiveness and Safety
Neurofeedback is a non-invasive technique that does not involve the use of medication or exposure to potential side effects. It is generally considered safe, with minimal adverse effects reported.
Personalized Approach
Neurofeedback can be tailored to the individual, targeting specific brain regions or frequencies based on their anxiety profile. This personalized approach allows for customized treatment plans that address the unique needs of each individual.
Neurofeedback Techniques for Anxiety
Neurofeedback can be delivered using various techniques and protocols depending on the specific needs of individuals with anxiety. Here are some commonly used neurofeedback techniques for anxiety management:
Alpha-Theta Neurofeedback
This technique focuses on enhancing the alpha and theta brainwave frequencies associated with relaxation and calmness. By training individuals to increase alpha and theta activity while reducing beta activity, alpha-theta neurofeedback aims to induce a state of deep relaxation and reduce anxiety symptoms.
SMR Neurofeedback
Sensory Motor Rhythm (SMR) neurofeedback targets the sensorimotor cortex, which plays a role in regulating emotional arousal. By increasing SMR activity in this region, individuals can learn to modulate their emotional responses, leading to a reduction in anxiety symptoms.
Beta Neurofeedback
Beta neurofeedback involves training individuals to regulate their beta brainwave activity, which is associated with arousal and alertness. By maintaining optimal levels of beta activity, individuals can learn to modulate their anxiety levels and promote a calmer state of mind.
Connectivity-Based Neurofeedback
This technique focuses on improving functional connectivity between brain regions involved in anxiety regulation, such as the prefrontal cortex and the amygdala. By training individuals to strengthen the communication between these regions, connectivity-based neurofeedback aims to enhance emotion regulation and reduce anxiety.
Neurofeedback Applications in Anxiety Management
Neurofeedback has shown promise in various applications for anxiety management. Here are some notable areas where neurofeedback has been utilized:
Generalized Anxiety Disorder (GAD)
Neurofeedback has been studied as a potential treatment for GAD, characterized by excessive worry and chronic anxiety. By training individuals to regulate their brainwave activity associated with anxiety, neurofeedback may help alleviate symptoms and improve overall well-being.
Panic Disorder
Panic disorder is marked by recurrent panic attacks and anticipatory anxiety. Neurofeedback techniques targeting specific brain regions, such as the amygdala and the prefrontal cortex, have shown potential in reducing the frequency and intensity of panic attacks and improving panic-related symptoms.
Social Anxiety Disorder
Social anxiety disorder involves intense fear and avoidance of social situations. Neurofeedback can be utilized to target brain regions involved in social cognition and emotional regulation, helping individuals modulate their anxiety responses in social contexts and improve social functioning.
Specific Phobias
Neurofeedback has been explored as a complementary intervention for specific phobias, such as fear of flying or heights. By training individuals to regulate their anxiety-related brainwave patterns, neurofeedback may contribute to desensitization and promote greater tolerance to phobic stimuli.
Performance Anxiety
Neurofeedback techniques can be beneficial for individuals experiencing performance anxiety, whether in academic, artistic, or athletic domains. By helping individuals regulate their anxiety responses and achieve a state of focused calmness, neurofeedback may enhance performance and reduce performance-related anxiety.
Considerations and Future Directions
While neurofeedback shows promise as a tool for anxiety management, there are several considerations and avenues for future exploration:
Individual Variability
Responses to neurofeedback can vary across individuals. Factors such as age, gender, baseline brain activity, and comorbid conditions may influence treatment outcomes. Understanding individual variability and developing personalized treatment protocols are essential for maximizing the effectiveness of neurofeedback.
Integration with Traditional Treatments
Neurofeedback can be utilized as a stand-alone treatment or integrated with other therapeutic approaches such as cognitive-behavioral therapy (CBT) or medication. Combining neurofeedback with traditional treatments may enhance the overall effectiveness of anxiety management strategies.
Long-Term Effects and Maintenance
The long-term effects of neurofeedback for anxiety management are still being explored. Continued research is needed to understand the durability of treatment effects and the maintenance of anxiety reduction beyond the training period.
Accessibility and Affordability
As neurofeedback technology advances, efforts should be made to improve accessibility and affordability. Developing user-friendly home-based systems or online platforms may broaden the reach of neurofeedback interventions and make them more accessible to individuals with anxiety.
Conclusion
Neurofeedback offers a promising approach for anxiety management by harnessing the brain's ability to self-regulate and reduce anxiety symptoms. Through techniques targeting specific brainwave patterns and functional connectivity, individuals can learn to modulate their anxiety responses and promote a calmer state of mind.
Research supports the effectiveness of neurofeedback in reducing anxiety symptoms across various anxiety disorders, including generalized anxiety disorder, panic disorder, social anxiety disorder, and specific phobias. By customizing neurofeedback protocols to address the unique needs of individuals, treatment outcomes can be optimized.
While neurofeedback holds promise, considerations such as individual variability, integration with traditional treatments, long-term effects, and accessibility need to be addressed. Continued research, technological advancements, and collaborative efforts will contribute to the further development and refinement of neurofeedback techniques for anxiety management.
Neuroenhancement through Transcranial Direct Current Stimulation (tDCS)
This non-invasive brain stimulation method involves the application of a weak electrical current to specific areas of the brain, with the aim of modulating neural activity and improving cognitive abilities. This article explores the concept of neuroenhancement through tDCS, its mechanisms of action, and its potential applications.
In the pursuit of cognitive enhancement and optimization, researchers have explored various techniques to enhance brain function. One such technique gaining attention is Transcranial Direct Current Stimulation (tDCS). This non-invasive brain stimulation method involves the application of a weak electrical current to specific areas of the brain, with the aim of modulating neural activity and improving cognitive abilities. This article explores the concept of neuroenhancement through tDCS, its mechanisms of action, and its potential applications.
Understanding Transcranial Direct Current Stimulation (tDCS)
To grasp the potential of tDCS as a neuroenhancement tool, it is important to understand the basics of this technique. Here are key points to consider:
Principles of tDCS
tDCS involves the application of a low-intensity electrical current, typically ranging from 1 to 2 milliamperes, to specific regions of the brain using electrodes placed on the scalp. The current flows between the anode (positive electrode) and the cathode (negative electrode).
Modulation of Neuronal Excitability
The electrical current in tDCS modulates neuronal excitability, influencing the firing rates of neurons in the targeted brain areas. The anode is associated with increased excitability (depolarization), while the cathode is associated with decreased excitability (hyperpolarization).
Non-Invasive and Safe
tDCS is a non-invasive and safe technique that does not require surgery or anesthesia. The low-intensity electrical current used in tDCS is well-tolerated by most individuals and has minimal side effects.
Mechanisms of Action in tDCS
The precise mechanisms through which tDCS exerts its effects are still under investigation. However, several mechanisms have been proposed to explain its influence on brain function:
Modulation of Resting Membrane Potential
tDCS modulates the resting membrane potential of neurons, making them more or less likely to fire action potentials. Anodal stimulation is believed to increase the excitability of neurons by depolarizing the resting membrane potential, while cathodal stimulation decreases excitability by hyperpolarizing the resting membrane potential.
Changes in Neurotransmitter Release
tDCS may influence the release and uptake of neurotransmitters in the stimulated brain regions. It has been suggested that tDCS can alter the balance of excitatory and inhibitory neurotransmitters, leading to changes in synaptic activity and neural communication.
Long-Term Potentiation and Depression
tDCS has been linked to the induction of long-term potentiation (LTP) and long-term depression (LTD), which are processes associated with synaptic plasticity. LTP strengthens synaptic connections, while LTD weakens them. Modulating these processes through tDCS may facilitate learning, memory formation, and cognitive enhancement.
Neurovascular Changes
tDCS can influence cerebral blood flow and oxygenation levels in the stimulated brain regions. These neurovascular changes may contribute to the observed effects of tDCS on cognitive function and performance.
Network Effects
The effects of tDCS extend beyond the stimulated brain region, impacting the connectivity and functional integration of neural networks. tDCS-induced changes in neural activity can propagate to distant brain areas, influencing their function and interaction within broader networks.
Understanding these mechanisms provides insight into how tDCS may enhance cognitive functions and optimize brain performance. In the next part of this article, we will explore the potential applications and benefits of tDCS in various domains.
Cognitive Enhancement with tDCS
One of the primary areas of interest in tDCS research is its potential for cognitive enhancement. Here are some key domains where tDCS has shown promise:
Working Memory and Attention
tDCS has been investigated for its ability to improve working memory and attention. Studies have demonstrated enhanced performance on working memory tasks and increased attentional focus following tDCS stimulation of the dorsolateral prefrontal cortex (DLPFC), a brain region implicated in these cognitive processes.
Language and Speech
Research has explored the use of tDCS to facilitate language and speech functions. Stimulation of the left hemisphere, particularly the Broca's area involved in speech production and the Wernicke's area involved in language comprehension, has shown potential for improving language fluency, word retrieval, and semantic processing.
Decision-Making and Executive Functions
tDCS has been investigated in the domain of decision-making and executive functions. Stimulation of the prefrontal cortex, involved in higher-order cognitive processes, has shown promising results in improving decision-making abilities, inhibitory control, and cognitive flexibility.
Learning and Skill Acquisition
tDCS has been explored as a tool for enhancing learning and skill acquisition. By stimulating brain regions relevant to specific tasks, such as the motor cortex for motor skill learning or the visual cortex for visual discrimination tasks, tDCS has been shown to facilitate skill acquisition and accelerate learning processes.
Neurorehabilitation and Clinical Applications
tDCS holds potential not only for cognitive enhancement but also for neurorehabilitation and clinical applications. Here are some notable areas where tDCS has shown promise:
Stroke Rehabilitation
tDCS has been investigated as a therapeutic intervention for stroke rehabilitation. By targeting the unaffected hemisphere or the lesioned area, tDCS can modulate cortical excitability and promote functional recovery in motor and cognitive functions.
Depression and Mood Disorders
tDCS has been studied as a treatment modality for depression and mood disorders. Stimulation of the dorsolateral prefrontal cortex has shown antidepressant effects, with research suggesting its potential as an adjunct to traditional treatment approaches.
Pain Management
tDCS has been explored for its analgesic effects in chronic pain conditions. By modulating pain perception and central pain processing, tDCS may offer a non-pharmacological approach to pain management.
Neurodevelopmental Disorders
tDCS research has extended to neurodevelopmental disorders such as autism spectrum disorder (ASD) and attention-deficit/hyperactivity disorder (ADHD). Preliminary studies suggest the potential of tDCS in modulating neural circuits and improving symptoms associated with these disorders.
Considerations and Future Directions
While tDCS holds promise as a non-invasive brain stimulation technique, there are considerations and future directions to be explored:
Individual Variability
Responses to tDCS can vary across individuals. Factors such as individual neurobiology, electrode placement, stimulation parameters, and inter-individual variability may influence the effects of tDCS. Personalized approaches and further research are needed to optimize individual outcomes.
Optimal Stimulation Parameters
The optimal stimulation parameters for tDCS, including electrode placement, current intensity, duration, and montages, are still being investigated. Understanding the optimal parameters for specific cognitive domains and clinical conditions is essential for maximizing the benefits of tDCS.
Safety and Ethical Considerations
While tDCS is generally considered safe, further research is needed to understand its long-term effects and potential risks. Ensuring the safety of participants and establishing ethical guidelines for tDCS research and application are important considerations.
Integration with Other Techniques
Exploring the combination of tDCS with other cognitive interventions, such as cognitive training or pharmacological approaches, may yield synergistic effects and enhance outcomes in neuroenhancement and neurorehabilitation.
Conclusion
Transcranial Direct Current Stimulation (tDCS) offers a non-invasive approach to enhance cognitive function, facilitate neurorehabilitation, and potentially treat various neurological and psychiatric conditions. By modulating neuronal activity, tDCS holds promise in cognitive enhancement, language and speech functions, decision-making, learning, and skill acquisition. Additionally, tDCS shows potential in neurorehabilitation for stroke recovery, depression, pain management, and neurodevelopmental disorders.
While tDCS research continues to evolve, careful considerations, such as individual variability, optimal stimulation parameters, safety, and ethical considerations, are vital. Further exploration of personalized approaches, optimal protocols, and integration with other techniques will contribute to advancing the field of tDCS and its applications.
As researchers continue to uncover the mechanisms and refine the application of tDCS, this non-invasive brain stimulation technique holds promise for improving cognitive abilities, supporting neurorehabilitation, and advancing our understanding of brain function.